Study by Rostand Idriss Tchana Tchamba, CHU – Amiens Sud,
Neurosurgery unit – Pr Michel Lefranc
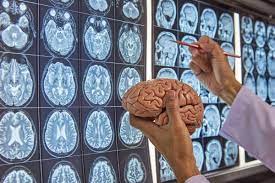
Internship Manager : Professor Michel LEFRANC
Head of the Service : Professeur Johann PELTIER
Reception Establishment : CHU Amiens – Picardie / Neurochirurgie
Introduction:
Electrophysiological treatments by deep brain stimulation are currently the only reference surgical treatments for the treatment of Parkinson’s disease at the stage of motor fluctuations, dystonia and drug-resistant essential tremor.
The electrode with the greatest therapeutic margin is selected to treat the patient, this being defined as the difference between the threshold for the appearance of a beneficial effect and the threshold for the appearance of an adverse effect secondary to stimulation . During the positioning of the electrode, a motor test is carried out in search of the threshold at which an undesirable motor effect appears. Several activated tissue volume (VTA) models have been described in the literature. The VTA makes it possible to virtualize the diffusion of the current delivered by the electrode. Our objective is to know if there is an anatomo-clinical correlation between the VTA, its diffusion to the pyramidal beam and the threshold of observed motor adverse effect.
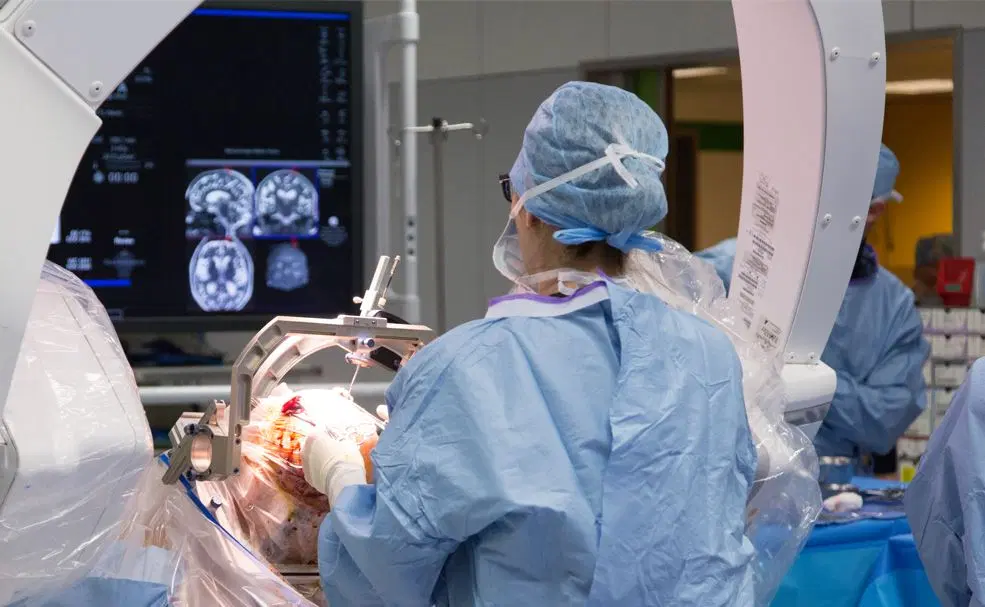
Method :
The study is retrospective, double-blind, monocentric, performed between September 2015 and August 2019. Preoperative imaging was performed under general anesthesia and included 3D T1 gadolinium, SWAN, FGATIR, and diffusion tensor imaging. (DTI) in 32 directions. The corticospinal tract is modeled from SteathlViz® (Medtronic). The VTA model is made from Suretune® software (Medtech). The operation is performed under general anesthesia and a motor test is performed during the positioning of the electrode. We have analyzed the intensity thresholds at which the VTA is located at the anatomical limit of the Internal Pallidum (VTA), the intensity thresholds at which the VTA touches the pyramidal tract, determined by the DTI (VTAdti) and we compared them to the thresholds of the perioperative adverse effects. A Pearson correlation test was performed.
Results :
A total of 18 patients were included in the study. Seven patients had morphologic imaging and quality DTI. No correlation was found between VTA values and the threshold for occurrence of intraoperative adverse events in the inner pallidum (p = 0.56). The AUV values were below the perioperative adverse event thresholds.
Conclusion :
VTA can not be used alone to predict the occurrence of the adverse event and replace an clinical motor test during surgery. The information delivered by the VTA is qualitative but could make it possible to optimize the positioning of the trajectory during the planning thereof.
INTRODUCTION :
Surgical electrophysiological techniques of cerebral stimulation are those that have currently proven their effectiveness for the treatment of symptoms of Parkinson’s disease at the stage of motor fluctuations but also essential tremor or drug-resistant dystonias (1–3). Deep brain stimulation aims to send an electrical current within a nucleus in order to obtain a therapeutic effect. The surgery consists of positioning electrodes within a target nucleus with a robot in stereotaxic condition.
The operation can be done under general or local anesthesia to keep connection with the patient and the surgery team and monitoring for other functions. The current is delivered by an electrode located in the target nucleus and connected to a subcutaneous generator. The mechanism of action of this deep brain stimulation is still unknown. There are hypotheses about antidromic and orthodromic activation of axons. During deep brain stimulation, axons have been shown to fire first. The initial part of the axon is the most excitable and the excitability of an axon is proportional to its diameter (4).
The electric potential and the second derivative of the electric potential, also called the activation function, are responsible for the activation of the axon (5). The second derivative of the electric potential is responsible for the depolarization and hyperpolarization of neurons located around the electrode (6)
During this operation, a motor clinical evaluation is carried out in order to determine the best positioning of the electrode defined by a wide therapeutic margin. This therapeutic margin is defined as the difference between the threshold at which the best clinical improvement of symptoms appears (akinesia, tremor, rigidity) and the threshold for the appearance of adverse effects (7) corresponding here to our therapeutic margin.
When the intervention is performed under general anesthesia, only the search for the adverse effect is evaluated. This undesirable effect may be manifested by oculomotor disturbances or by tonic muscular contraction of a part of the hemibody contralateral to the stimulation. The appearance of this muscular contraction is secondary to the activation of the axons of the corticospinal bundle by the diffusion of the electric field to the internal capsule
No current study provides preoperative predictions of the amplitude of the current from which an undesirable motor effect will appear in proportion to the diffusion of the current in the internal capsule; the search for the adverse effect is therefore carried out intraoperatively
for the optimization of the positioning of the final electrode and to ensure that the assigned voltages give the desired therapeutic effect without however causing undesirable effects in the patient.
Models of the diffusion of the electric field delivered by the stimulation electrode exist. The activation of the axons is not limited to the target area by the diffusion of the electric field (6,8). The volume of activated tissue (VTA) consists of the virtual reproduction of the diffusion of the current to the anatomical structures close to the chosen target. The activation function is used in different models to estimate the volume of activated tissue (8).
The diffusion of the electric field depends on the location of the electrode (6), its geometry (9) and the electrical properties of the surrounding tissues (10).
The electrical properties of tissues modifying the diffusion of the electric field include: orientation (11), fiber diameter and conductivity (8). The amplitude of the current and the duration of the pulse are important determinants of the intensity of the electrical charge emitted and therefore of the appearance of side effects to stimulation (7).
Until now, no correlation between the VTA in the Gpi and an intraoperative motor clinical effect has been demonstrated, this because the virtual estimation of the electric field is made approximately electronically and the imaging has limits. . Presumably, this is due to the limit of the virtualization of the diffusion of the electric field which is only an extrapolation from algorithms as well as to the quality limits of the imagery.
However, there are improvements in imaging techniques allowing better visualization of the anatomical structures and new models virtualizing the VTA: the Fast Gray Matter Acquisition T1 Inversion Recovery (FGATIR) sequence which makes it possible to visualize the pallidum and in particular the internal Globus pallidum (12), the high-resolution 3-dimensional T2*-weighted angiography (SWAN) sequence which makes it possible to visualize the subthalamic nucleus (13) and diffusion tensor imaging in 32 directions which makes it possible to obtain tractography of the white matter fibers.
The objective of our study is to know if there is an anatomo-clinical correlation between the volume of activated tissue (VTA), and pathologies of movement. The demonstration of such a correlation would make it possible to dispense with the intraoperative motor test. Determining this correlation would also be of interest when planning the target by optimizing the objectives of electrophysiological techniques and reducing the operating time and therefore the risk of infection.
TOOLS AND METHODOLOGY :
Patients:
Our study is a double-blinded retrospective study.
Patients who received stimulation of the GPI between September 2015 and July 2019 were systematically included.
The pathologies treated were abnormal movements related to Parkinson’s disease and primary dystonia. The target nuclei were those of the internal globus pallidum (Gpi).
The choice of the target therapy was determined before the intervention after carrying out an operability assessment and a multidisciplinary consultation. For our study after collecting patient data, we had 35 patient files to study, of the files provided, 22 files corresponded to Gpi stimulations, and after analysis of the files 4 were excluded because the electrophysiological data were unavailable or not usable; 18 patients, aged 19 and 79, were therefore included in our study, all having undergone their intervention in the neurosurgery department of the CHU-Amiens Sud (Salouël).
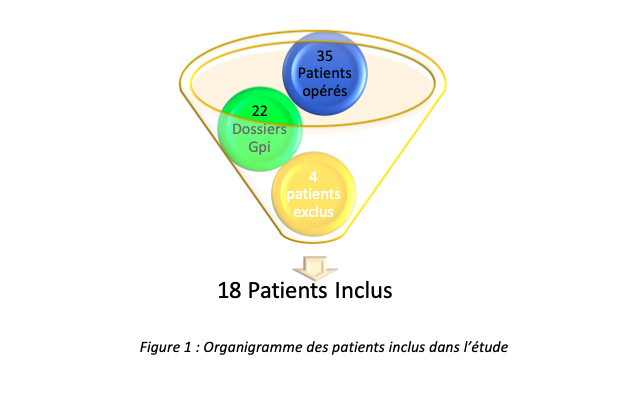
Electrophysiology:
During the operation, the patient is under general anesthesia, supine position. Fixed head on frame Leksell itself to the stereostatic robot. Six trans-osseous ficidaries are placed transcutaneously; then the O’arm is put in place and a 3D scanner which will serve as reference imaging is carried out, we carry out a motor test. Sedation is monitored by EEG. From this EEG, a bispectral index (BIS) is extracted. This index assesses the degree of synchronization of the EEG tracing, which increases with the depth of anesthesia. The anesthetic agents used are sevoflurane, a halogen gas, and alfentanil (14). Intraoperative motor testing is performed by monopolar stimulation with the definitive macro-electrode type 3389 from Medtronic®. The reference is subcutaneous, located at the level of the torso. We perform a high frequency stimulation (130Hz), with a pulse duration of 100μs by gradually increasing the stimulation amplitude in steps of 0.5 mA. Tests on pads 1 and 2 are carried out systematically. Plot 1 is the plot located within the predetermined target. Depending on the test results, plots 0 and 3 can also be tested; for our study, pins one and two were retained in most patients, however according to the data, only pin 1 was retained in 6 patients. If the motor testing finds an adverse effect threshold below 3.0 mA, then the electrode is repositioned and a new motor testing is performed.
Imaging :
Before the deep brain stimulation operation, a brain MRI and a brain scan Stereotaxic examination under general anesthesia is performed on the patient.
The brain scan is performed by taking sections of the patient’s entire head with a matrix of 512512, continuous images, 0.625 mm thick. The MRI sequences performed were the 3D T1 gradient echo sequence after injection of gadolinium (3D T1 Gado) with a 512512 matrix, continuous images 1 mm thick; the high resolution SWAN sequence in 3 dimensions (512512 matrix, continuous image 1mm thick); the sequence FGATIR; and 32-direction diffusion tensor sequences (176176 array with 2mm slice thickness).
Diffusion tensor imaging (DTI) analyzes the diffusion of water within white matter. This diffusion of water is anistropic, facilitated along the fibers and decreased perpendicular to the fibers. The diffusion tensor makes it possible to determine for each voxel of the image, the preferential orientation of the water molecules. This orientation of the water molecules is determined from the measurement of the diffusion coefficient obtained from at least 6 directional gradients (15). The tractography will build the fiber bundle from the orientation information of the known water molecules in each voxel previously defined by the diffusion tensor.
Several images by OARM ® flat sensor scanner are performed during the operating procedure to see the positioning of the electrode. Imaging parameters are one of the imaging are 512*512 matrix, continuous images with 0.8mm thickness.
Softwares :
In our study, we mainly used two software programs: DxCare® software and Suretune® software; other software such as Zotero® were also used for documentation.
DxCare®
Dxcare is an optimized management software for patients in hospital structures with several functionalities including the collection of acts and coding in the patient’s file; streamlining and optimizing the performance of medical and financial personnel by streamlining information and centralizing data within a single database.
For our study, it was mainly a question in this software of accessing the circuit of the various patients having undergone an operative intervention of cerebral stimulation of the Internal Globus Pallidus (Gpi) and of collecting in the operative report the patients the intensities responsible for the adverse effects. intraoperatively.
Suretune®
The SWAN, FGATIR, 3D T1 Gado, DTI sequences of the pyramidal beam and the intraoperative OARM® acquisition, showing the positioning of the electrode, were merged in the Suretune® software (Figure 2).
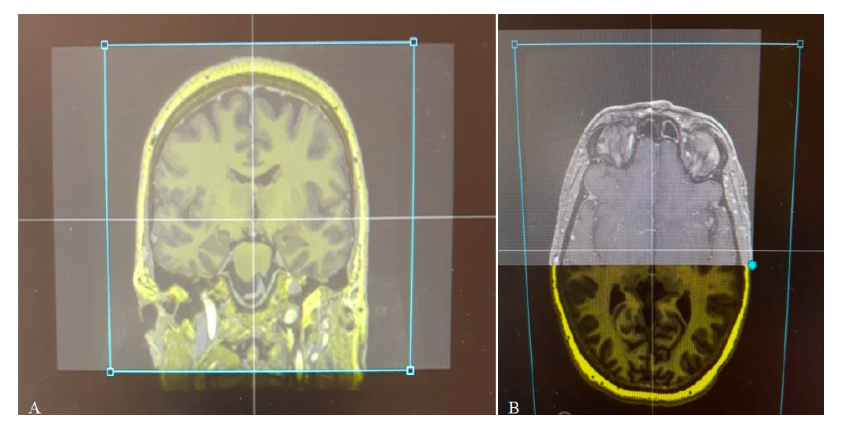
This software models the VTA from a modeling of an axon, the electrode, the delivered wave. The axon model notably takes into account the geometry (its diameter, the diameter of the node of Ranvier and the distance between 2 nodes of Ranvier) and the membrane conduction of the axon. The Medtronic® 3389 electrode model is virtualized to simulate current diffusion. It is this electrode model that we use for deep brain stimulation procedures. Finally, the tissue surrounding this electrode is considered homogeneous with an impedance of 1 kΩ. (16).
This virtualization model of the VTA has been compared to other models described in the literature finding similar results for estimating the VTA (6,17,18).
This software will make it possible to virtualize the VTA according to stimulation parameters that we define and which include the intensity (mA), the frequency (Hz), the pulse duration (μs), and the diameter of the axon activated (μm). The stimulation parameters used to virtualize the VTA corresponded to the intraoperative stimulation parameters, i.e. a pulse duration of 100μs and a frequency of 130Hz. The diameter of the activated axon was considered to measure 3μm.
The determination of the intensity threshold of the VTA, which would be responsible for an undesirable motor effect by damage to the pyramidal bundle, was done postoperatively by an examiner who was unaware of the threshold of undesirable effect observed intraoperatively during motor testing.
We have determined two intensity thresholds in mA at which we assume that the VTA is responsible for the appearance of an adverse motor effect. We looked for the stimulation intensity threshold from which the VTA touched the anatomical limit of the nucleus (VTAn) and the stimulation intensity threshold when the VTA touched the pyramidal bundle, determined by tractography (VTAdti).
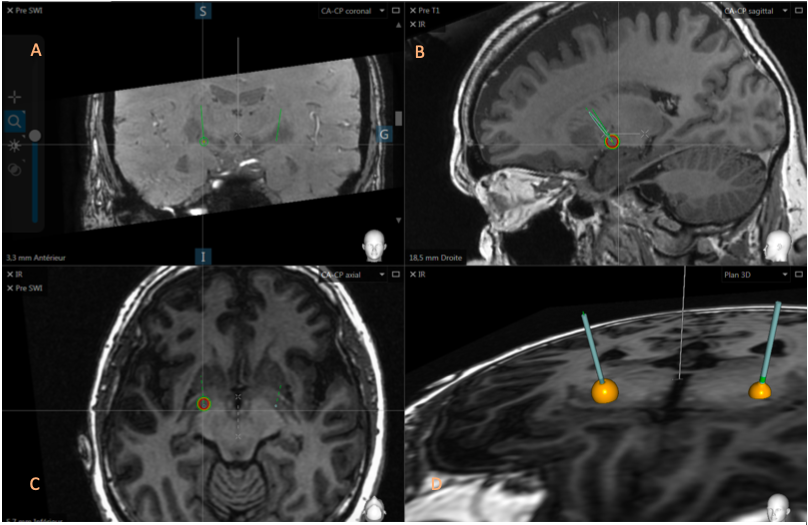
We measured the stimulation intensity threshold of the VTA at the anatomical limit of the nucleus assuming that when the VTA exits the nucleus at the level of the posterior arm of the internal capsule or laterally with respect to the NST, the VTA would then be in contact with the pyramidal beam and it would be responsible for an undesirable effect.
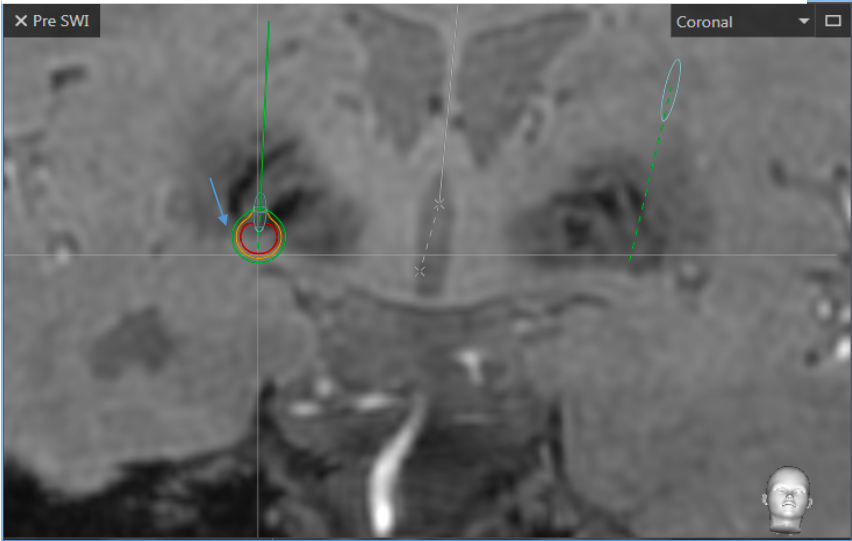
We chose to measure the VTA at this level since some patients did not have interpretable tractography of the pyramidal tract.
Figures 3 and 4 illustrate a VTA affecting the pyramidal bundle, the different cuts, coronal, axial, sagittal and the 3D plane allow a better observation of the deployment of the pyramidal bundle.
Statistical Analysis :
We performed a correlation test between the value of the stimulation intensity threshold from which the VTA is at the anatomical limit of the Internal Pallidum and the intensity thresholds responsible for intraoperative adverse effects.
We also performed a Bravais Pearson correlation test between the value of the stimulation intensity threshold from which the VTA touches the pyramidal bundle, determined by tractography, and the intensity thresholds responsible for intraoperative adverse effects.
Results :
In our data analysis, we have p=0.56; we carried out a correlation test between the value of the stimulation intensity threshold from which the VTA is at the anatomical limit of the nucleus and the intensity thresholds responsible for intraoperative adverse effects which shows us a very weak correlation between the two elements, an illustration of the analysis table can be seen in figure 5.
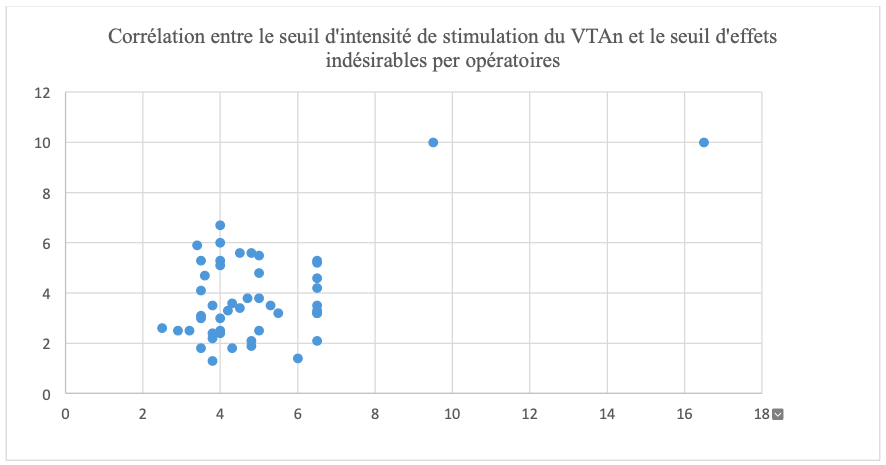
DISCUSSION :
In our study, we sought to highlight a significant correlation between the value of the stimulation intensity threshold from which the VTA is located in the Internal Pallidum and the thresholds of intraoperative motor adverse effects. This is the first study of its kind showing a correlation between intraoperative clinical findings and a prediction of clinical effect based on electric field virtualization with Suretune® software.
However, our results showed a minimal correlation between the data, with a strong dispersion of these. This therefore makes it impossible to use the VTA alone to estimate an adverse effect in clinical practice.
The presence of this weak correlation may be secondary to the VTA model proposed by the Suretune® software, to fusion errors between the preoperative and intraoperative images, or to errors in the reconstruction of our statistical series. The need for an intraoperative test is therefore always necessary in order to determine the limit of the occurrence of adverse effects.
CONCLUSION :
We observed that there is a minimal correlation between the volume of activated tissue, its diffusion to the pyramidal bundle and the appearance of intraoperative motor adverse effects in the Internal Pallidum.
This is the first study showing a relationship between the threshold values of stimulation intensity from which the VTA is located at the anatomical limit of the nucleus and the thresholds of intraoperative adverse effects with the Suretune® software. However, this correlation remains weak, making it impossible to use the VTA as a predictor of the appearance of motor adverse effects and still requiring motor testing. We have also shown that the values of the stimulation intensity threshold from which the VTA is located at the anatomical limit of the nucleus are lower than the threshold for the appearance of intraoperative adverse effects.
The information from the VTA is therefore qualitative. VTA can be used to improve targeting during trajectory planning, but we cannot dispense with intraoperative clinical testing. The improvement of imaging tensor diffusion sequences and virtualization models of the VTA will perhaps make it possible to demonstrate a strong correlation between the VTA and the intraoperative occurrence of adverse effects, thus making it possible to dispense with testing. intraoperative motor.
The improvement of the imagery must be able to improve the spatial resolution, decrease the distortions of the images and increase the number of gradients. The improvement of the VTA models must include an axon model corresponding to the axons of the pyramidal bundle, taking into account the inhomogeneity of the tissue surrounding the electrode, and the anisotropy of the medium
References :
- Benabid AL, Pollak P, Gao D, Hoffmann D, Limousin P, Gay E, et al. Chronic electrical stimulation of the ventralis intermedius nucleus of the thalamus as a treatment of movement disorders. J Neurosurg. 1996 Feb;84(2):203–14.
- Vercueil L, Pollak P, Fraix V, Caputo E, Moro E, Benazzouz A, et al. Deep brain stimulation in the treatment of severe dystonia. J Neurol. 2001 Aug;248(8):695–700.
- Deep-Brain Stimulation for Parkinson’s Disease Study Group, Obeso JA, Olanow CW, Rodriguez-Oroz MC, Krack P, Kumar R, et al. Deep-brain stimulation of the subthalamic nucleus or the pars interna of the globus pallidus in Parkinson’s disease. N Engl J Med. 2001 27;345(13):956–63.
- Carron R, Chabardès S, Hammond C. [Mechanisms of action of high-frequency deep brain stimulation. A review of the literature and current concepts]. Neurosurgery. 2012 Aug;58(4):209–17.
- Rattay F. Analysis of models for external stimulation of axons. IEEE Trans Biomed Eng. 1986 Oct;33(10):974–7.
- McIntyre CC, Mori S, Sherman DL, Thakor NV, Vitek JL. Electric field and stimulating influence generated by deep brain stimulation of the subthalamic nucleus. Clin Neurophysiol Off J Int Fed Clin Neurophysiol. 2004 Mar;115(3):589–95.
- Lefranc M, Zouitina Y, Tir M, Merle P, Ouendo M, Constans J-M, et al. Asleep robot-assisted surgery for the implantation of subthalamic electrodes provides the same clinical improvement and therapeutic window as awake surgery. World Neurosurg. 2017 Jul 19;
- Sotiropoulos SN, Steinmetz PN. Assessing the direct effects of deep brain stimulation using embedded axon models. J Neural Eng. 2007 Jun;4(2):107–19.
- Butson CR, McIntyre CC. Role of electrode design on the volume of tissue activated during deep brain stimulation. J Neural Eng. 2006 Mar;3(1):1–8.
- Grill WM. Modeling the effects of electric fields on nerve fibres: influence of tissue electrical properties. IEEE Trans Biomed Eng. 1999 Aug;46(8):918–28.
- Ranck JB. Which elements are excited in electrical stimulation of mammalian central nervous system: a review. Brain Res. 1975 Nov 21;98(3):417–40.
- Sudhyadhom A, Haq IU, Foote KD, Okun MS, Bova FJ. A high resolution and high contrast MRI for differentiation of subcortical structures for DBS targeting: the Fast Gray Matter Acquisition T1 Inversion Recovery (FGATIR). NeuroImage. 2009 Aug;47 Suppl 2:T44-52.
- Lefranc M, Derrey S, Merle P, Tir M, Constans J-M, Montpellier D, et al. High-resolution 3-dimensional T2*-weighted angiography (HR 3-D SWAN): an optimized 3-27
17
T magnetic resonance imaging sequence for targeting the subthalamic nucleus. Neurosurgery. 2014 Jun;74(6):615–626; discussion 627 - Fluchere F, Witjas T, Eusebio A, Bruder N, Giorgi R, Leveque M, et al. Controlled general anesthesia for subthalamic nucleus stimulation in Parkinson’s disease. J Neurol Neurosurg Psychiatry. 2014 Oct;85(10):1167–73.
- Oppenheim C, Ducreux D, Rodrigo S, Hodel J, Tourdias T, Charbonneau F, et al. [Diffusion tensor imaging and tractography of the brain and spinal cord]. J Radiol. 2007 Mar;88(3 Pt 2):510–20.
- Åström M, Diczfalusy E, Martens H, Wårdell K. Relationship between neural activation and electric field distribution during deep brain stimulation. IEEE Trans Biomed Eng. 2015 Feb;62(2):664–72.
- Kuncel AM, Cooper SE, Grill WM. A method to estimate the spatial extent of activation in thalamic deep brain stimulation. Clin Neurophysiol Off J Int Fed Clin Neurophysiol. 2008 Sep;119(9):2148–58.
- Madler B, Coenen VA. Explaining clinical effects of deep brain stimulation through simplified target-specific modeling of the volume of activated tissue. AJNR Am J Neuroradiol. 2012 Jun;33(6):1072–80.
- Mathai A, Wichmann T, Smith Y. More than meets the eye-myelinated axons crowd the subthalamic nucleus. Mov Disord Off J Mov Disord Soc. 2013 Nov;28(13):1811–5.
- Ashby P, Kim YJ, Kumar R, Lang AE, Lozano AM. Neurophysiological effects of stimulation through electrodes in the human subthalamic nucleus. Brain J Neurol. 1999 Oct;122 (Pt 10):1919–31.
- Bertrand A, Oppenheim C, Moulahi H, Naggara O, Rodrigo S, Patsoura S, et al. [Diffusion-weighted imaging of the brain: normal patterns, traps and artifacts]. J Radiol. 2006 Dec;87(12 Pt 1):1837–47.
- Coenen VA, Fromm C, Kronenbürger M, Rohde I, Reinacher PC, Becker R, et al. Electrophysiological proof of diffusion-weighted imaging-derived depiction of the deep-seated pyramidal tract in human. Zentralbl Neurochir. 2006 Aug;67(3):117–22.
- Coenen VA, Jenkner C, Honey CR, Mädler B. Electrophysiologic Validation of Diffusion Tensor Imaging Tractography during Deep Brain Stimulation Surgery. AJNR Am J Neuroradiol. 2016 Aug;37(8):1470–8.