by Dr Rostand Idriss Tchana, Pharmacist
Resume
Drug delivery system have been developed past years improved and tried to improve the best security and care for the patients. There is a lot of technologies involved in this process as the industry raised several investments in nanomaterials (Shuang et al., 2017).
The impact of these different improvements in patient care leads to specific intelligent biomaterials designed to release in response to either physical, chemical or biological triggers. The specific use of this DDS leads to spatiotemporal control and deeply optimize the drug effects (Carlos M. et al., 2019).
Mostly the strategies are using drug conjugated to the precarrier (prodrug), in an inactive state who’s degraded in-vivo and deliver on target the active agents. The unique structure of the produgs showed self-assembly properties with nanoparticles with nanoparticles through chemical modifications. Also several advantages been present on them including high efficiency, amelioration of drug availability, drug release controllability and less tendency to agglomerate after encapsulation ( Luo C. et al., 2014). After consideration, we can devide the DDS responsive to some stimuli into two categories addressed as internal (endogenous) responsive DDS and external (exogeneous) responsive DDS.
It’s really obvious for us as pharmacists to have a bibliographical study on the topic and check the late advances in the topic and what improvements can be done to have an optimistic innovation and best care for the patients.
Introduction
Drug delivery systems and galenic formulation technologies are the most important concerns of the pharmaceutical industry. It’s really important to have the drug delivered to the targeted site and avoiding non-targeted sites relying on adverse effects and also toxicity.
The latest advancements in DDS technologies Currently, lead to improvements in nanomedicines in cancer and several pathologies such as lymphoma, leukemia, inflammation etc. Different technologies merged in this field as liposomes and micelles (Liu et al., 2019), built up as polymer nanoparticles, with the significant advancement of nanotechnology, a variety of nanomedicines have been developed as polymer nanoparticles (Shuang et al., 2017), inorganic nanocrystals (Yang G. et al., 2017), biomimetic nanomaterials (Ouyang J. et al, 2018).
The different elements integrated into the Stimuli-responsive DDS can be divided into two big types depending on the stimuli type used : internal stimuli using the tumor micro-environment (TMO) (e.g., Redox environment, pH, enzymes and hypoxic etc.) and external stimuli not in the tumor environment but induced by the therapist voluntarily (e.g., light, thermo, ultrasound, and magnetism field, etc.) (Anel Xie et al., 2020).
The prodrug (drugs conjugated to the precarrier), is inactive after administration and can be degraded in vivo to gain the required efficiency, this with a simple modification of its structure. This system gives several advantages as higher bioavailability, better efficiency, control on the release (target), and less risk of agglomeration after encapsulation ( Luo C. et al, 2014).
It is now obvious and relevant to discuss the importance of prodrug-based nanomedicines specified in the sensitive respond to certain stimuli for drug delivery systems (DDS).
Context
As we’re in an evolving world where the purpose is to give the best care to the patients with an optimistic balance risks/benefits, drug delivery on the target site with minimal toxicity to the organs that are not supposed to be targeted remains a significant challenge in modern drug delivery technologies. Past years, advancements such as combinatory chemistry, biotechnology, gene-based drugs, and high throughput screening provide several new molecular entities for various diseases, but with many of these drugs, the optimal treatment is only possible if sufficient delivery of the compounds to the target site of action. Also, treatments with biological drugs, such as DNA and proteins, may suffer from deficient drug delivery to the target cells (V.P. Torchilin, 2018).
Nanomedicine drug delivery has recently received tremendous attention in the treatment of various diseases such as breast and ovarian cancer (Sankha and al. 2022). Drug delivery to the target tissues such as head and neck cancer and even infected regions of the body, brain and back eye segment is challenging due to the high difficulty to reach the right concentration amount of the active principles in those regions (Ishwor Poudel and al. 2022). Therefore, the need for localized and targeted delivery is becoming increasingly important in reducing toxicity and side effects, increasing the drug efficacy with a decrease of dose and even medicines costs. The nonspecific delivery of drugs and tissue biodistribution and rapid drug metabolism or excretion from the body makes the drug action less efficient and requires increased dose witch causes a real challenge for drug design and the pharmaceutical industry.
Thus the understanding of molecular aspects associated with different pathologies has led to deep understanding of endogenous and exogeneous material that can be exploited to devise “stimuli responsive” material that can be targeting specific sites of application into the body. These are new targets of the pharmaceutical industry regarding chronic diseases that can be exploited need to develop new DDS and mainly SRD are the main target in new pharmaceutical strategies and the industry is investing more and more in these concepts.
Stimuli responsive drugs
Stimuli responsive drugs are one of the latest innovation in therapeutic and drug delivery and may constitute an ideal and innovative approach to have more results with less side effects in several treatments.
Stimuli responsive drugs are drugs made out of material that can modify reversibly or not their physical properties in response to an internal or external stimuli, causing change in their particles properties such as dimensions, structure and interactions, which leads to rearrangements on their aggregation state where the responsiveness is supplied from a part of the material.
There are different therapeutical applications for SRD, most used are in oncology where the latest innovation were the use of MSR-DDSs are a fine touch and offer a good platform for co-delivering of agents and constitute a really interesting spot to avoid multidrug resistance (Jia R and al., 2021).
There is different classification to SDR due to the type of material they’re made of and also the type of stimuli they’re responsive to : Internal or external stimuli responsive drugs depending on the location of the stimuli element chosen, and depending of the type of stimuli we can have pH, light, ultrasound or redox stimuli used. Therefore we can manage to match them into different ways to represent SDR (fig. 1) where we do have in blue external stimuli responsive drugs and in red internal stimuli responsive drugs.
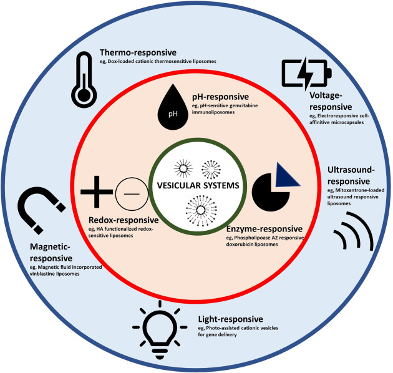
Some Definitions
Drug delivery system :
Drug delivery system are the system used to deliver drugs / active agents to the target sites of the pharmacological agents.
To reach their target in the body, drugs can be inserted following different types of route depending on the active principle and the target routes of the drug itself. These routes can be classified as depending on their “starting point” ( Laura Elizabeth Lansdowne, Technology Networks / Drug Discovery, December 2020).
Thus we do have the following delivery routes that can be used to our drugs to reach targets in the body (fig 2) :
Buccal drug delivery
Buccal drug delivery corresponds to the administration of a drug through the buccal mucosa (lining of the cheek) (Jinsong Hao et al. , 2003) . This delivery route avoids gastrointestinal degradation process and first-pass effect (rapid drug uptake and metabolism into inactive compounds by the liver), there is a real “challenging” barrier to drug absorption through this route, especially for larger biopharmaceuticals – delivery is currently limited to small molecule drugs with lipophilic properties as they can readily cross the membrane (Flavia Laffleur, Drug Development and Industrial Pharmacy, 2014 ). The use of buccal route for drug delivery is often for extended-release drug delivery (Drug release on an extended time period with a controlled process), A deep connection and adhesion/attachment to the mouth mucosa are mostly preferred. Different formulations have been developed for buccal delivery including; tablets, gels, lozenges and patches (Jinsong Hao et al. , 2003).
Nasal drug delivery
Nasal drug delivery involves the delivery of a drug through the nose with a connection with the nasal cavity. Nasal spray medications are usually used for the treatment ofpathologies affecting the upper respiratory tract (e.g., nasal congestion, allergic rhinitis) ( Karim Amighi, Galenic Pharmacy course, 2021 – 2022). We can also in some circumstances use this this route for a systemic target for example regarding the delivery of small molecules drugs – e.g., the migraine medication olmatriptan (Zomig®) (CBIP, consultation le 26/07/2022).The great vascularization of this mucosa helps to increase access to blood circulation for drugs and avoidance of first-pass metabolism.
Ocular drug delivery
Ocular drug delivery is a tricky route for the scientists ant the patients as well. This is due to the unique anatomy and physiology of this organ having a static, dynamic and metabolic ocular barriers impede the absorption of drugs through the eye. This organ can use different targets of drugs delivery with a specific approach. Different approaches and innovative works helped passing challenges of delivering drugs to ocular tissues through identification of different transporters (efflux and influx transporters) and modification of drugs technology formulation to target these transporters in the eye and modifying drugs to target these transporters (Gaudana et al. 2010).
Oral drug delivery
The most common route used for drug is the oral drug delivery, several key points justifies this use as a way of drug administration as non-invasive nature, ease of use, cost-effectiveness and the highly absorptive properties of the gastrointestinal (GI) tract. are Oral drug delivery is by far the most well-known and often the preferred route of drug administration due to its non-invasive nature, ease-of-use, cost-effectiveness, and the highly absorptive properties of the gastrointestinal (GI) tract ( CBIP, 2022). Several conditions are required for the oral delivery to be successful, the aqueous solubility of the drug compound in the GI system as well to make sure if there is any modification to the drug technology to be added for increase of the bioavailability. This route requires particular attention of caregivers and health practitioners for specific patients populations including; pediatric, geriatric and those with cognitive impairment.
Pulmonary drug delivery
For this delivery route, the inhalation through the mouth and into the airways is required. These can be used for treating local diseases in the lungs. The effects of this drug technology use can be for a local or systemic effect. Last advancements in science helped to move forward with pulmonary drug delivery as a potential route of administration for systemic diseases regarding the vast absorption potential due to the large absorptive surface area and the great permeable membrane of the alveolar region. This route is also a great space due to the unaffected value by dietary and interpatient variability ( Labiris NR et al., 2003).
Sublingual drug delivery
This route of drug delivery is refereeing for drug administration under the tongue, then absorption into the bloodstream via the tongue’s ventral surface and the mouth floor is observed. of the mouth ( Narang et al. , 2010) . The fast absorption of sublingual route helps to set rapid drug effect. Sublingual drug delivery system also avoids hepatic first‐pass metabolism effect. Somehow, patient’s compliance can be affected because of interaction with talking, eating and drinking where they can cause disruption. In addition, absorption and consequently efficacy of the drug is reduced by smoking due to vasoconstriction of the vessels, therefore use in smokers is not recommended (Hao Zhang et al., 2002).
Transdermal drug delivery
Transdermal drug delivery refers to a DDS method for systemic effect by applying the formulation directly onto intact skin ( of delivering a drug systemically by applying a formulation onto intact skin ( Alkilani et al., 2015). The trajected way used by the drug with this route is first penetration through the stratum corneum and then progression through deep epidermis and dermis and absorption via microcirculation. The non-invasive characteristic of this route can help for patients unconscious or suffering from regurgitations ( Praunitz et al., 2004).
Vaginal/anal drug delivery
Anal and vaginal drug have a faster onset of action compared to the oral route and a higher bioavailability. Their use could be for local or systemic effect depending on the targeted pathology. First-pass metabolism is avoided and no interactions with gastrointestinal disturbances is observed (Wolfson et al., 2000).
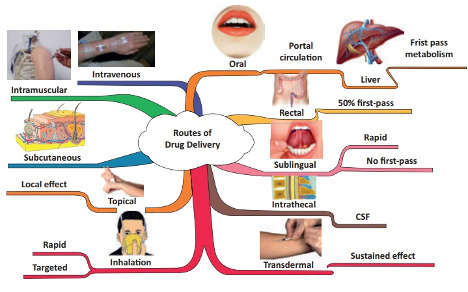
Stimuli Responsive drugs :
Stimuli responsive drugs are drugs that responds to a specific trigger to deliver their cargo on the specific site targeted. Their use is mostly to overcome adverse drug effect and optimize efficiency depending on the route of administration ( Rahim MA et al., 2021). We can summarize the different effect of the SRD strategies on the figure below.
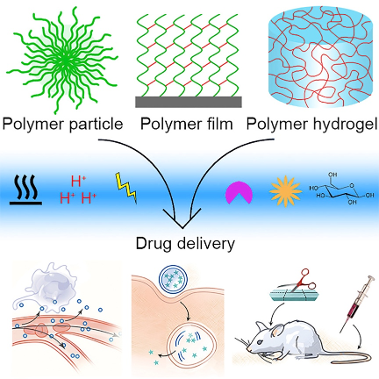
Internal Stimuli responsive drugs
Internal stimuli responsive drugs are drugs made out of smart biomaterials that respond to triggers present in their microenvironment such as pH or specific enzymes ( Wells CM et al., 2019).
External Stimuli responsive drugs
External stimuli responsive drugs concerns drugs that are made with smart biomaterials responding to triggers present out of their microenvironment such as electromagnetic field, light or even acoustic stimulaton ( Wells CM et al., 2019).
Multi-Stimuli responsive drugs
Multi-Stimuli responsive drugs are drugs made with biomaterials that responds to double or triple triggers depending on the formulation. These materials are engineered to have high sensibility to the surrounding physiological environments and release the encapsulated cargo with a deep specificity to their target (Xiao Fu et al, 2018).
Therapeutical Applications
In DDS, stimuli responsive drugs are used for different pathologies but mostly tumors are the one targeted,the use of these materials can be shortened in the table below regarding the use of nanocarriersin vesicular DDS ( table 1)
Table 1 Different Vesicular systems under clinical trial with applied filters : active ( not recruiting), Interventionnal Studies ( Clinical trials) at clinicaltrials.gov Other sources : H. He, L. Liu, E. E. Morin, M. Liu, A. Schwendeman. Survey of clinical translation of cancer – nanomedicines – lessons learned from successes and failures Acc Chem Res 2019 52(9):24452461 AC Anselmo, S Mitragotri Nanoparticles in the clinic : any update Bioeng Transl Med 2019 ,4 ( 3) : e10143 DJ Crommelin, P Van Hoogevest G Storm The role of liposomes in clinical nanomedicine development What Now ? now What? J Controlled Rel, 2020 318:256263
Vesicular system | Commercial products or clinical trials | Applications (approved) |
Doxil | Doxil/FDA approved (1995) | Breast cancer, Ovarian cancer |
Doxorubicin liposomes (Pegylated) | Caelyx/EMA approved (1996) | HIV-associated Kaposi’s sarcoma |
Daunorubicin liposomes (Pegylated) | DaunoXome/FDA approved (1996) | HIV-associated Kaposi’s sarcoma |
Paclitaxel liposomes | Lipusu/Approved in China (2003) | Metastatic gastric cancer |
Cytarabine liposomes | Depocyt/Approved in USA (2006) | Leukemia |
Paclitaxel micelles | Genexol-PM/Approved in Korea (2007) | Breast cancer |
Mifamurtid liposomes | MEPACT/EMA approved (2009) | Osteosarcoma (Primary) following surgery |
Irinotecan liposomes (Pegylated) | Onivyde/FDA approved (2015) | Metastatic pancreatic cancer (secondary) |
Cytarabine:Daunorubicin, (5:1) liposomes | VYXEOS CPX-351/FDA approved (2017) | Acute myeloid leukemia |
Propofol liposomes | Diprivan/FDA approved (1989) | Induction of anesthesia |
Amphotericin-B liposomes | AmBisome/FDA approved (1997) | Cryptococcal meningitis in HIV-infected patients Systemic fungal infections Visceral leishmaniasis in immunocompromised patients |
Verteporfin liposomes | Visudyne/FDA approved (2000) | Age-related macular degeneration |
Doxorubicin liposomes (Pegylated) | Doxil/Caelyx: 241# | Solid malignancies, ovarian, breast cancer, luekemia, lymphomas, prostate, metastatic or liver cancer |
Daunorubicin liposomes | Daunoxome: 48# | Leukemia |
Doxorubicin liposomes | Myocet: 22# | Breast cancer, lymphoma and ovarian cancer |
Paclitaxel liposomes | Lipusu: 1# | Breast and nonsmall cell lung cancer |
Cytarabine liposomes | Depocyt: 11# | Solid tumors, Lymphoma |
Mifamurtide liposomes | Mepact: 1# | Osteosarcomas |
Vincristine liposomes | Marqibo: 149# | Cancers including lymphoma, brain, leukemia, melanoma |
Irinotecan liposomes (Pegylated) | Onivyde: 7# | Solid Malignancies, breast cancer, pancreatic cancer, sarcomas |
Cytarabine:Daunorubicin (5:1) liposomes | Vyxeos: 2# | Leukemias |
Propofol liposomes | Diprivan: 19# | General anesthesia in morbidly obese patients, open heart surgery, spinal surgery |
Amphotericin-B liposomes | Ambisome: 7# | Invasive fungal infections |
Verteporfin liposomes | Visudyne: 1# | Macular degeneration |
Cisplatin (Pegylated) liposomes (Lipoplatin) | Phase-3 | Nonsmall cell lung cancer |
Paclitaxel liposomes (EndoTAG-1) | Phase-3 | Breast cancer Pancreatic adenocarcinoma |
Doxorubicin liposomes HER2 targeted (MM-302) | Phase-3 | Breast cancer |
Doxorubicin liposomes (thermally sensitive) (ThermoDox) | Phase-3 | Hepatocellular carcinoma |
VCL-1005 plasmid liposomes (Allovectin-7) | Phase-3 | Melanoma |
MUC1 antigen liposomes (Tecemotide) | Phase-3 | Nonsmall cell lung cancer |
Paclitaxel micelles (NK105) | Phase-3 | Breast cancer |
Cisplatin micelles (NC-6004) | Phase-3 | Pancreatic cancer |
Irinotecan and floxuridine liposomes (CPX-1) | Phase-2 | Colorectal cancer |
Bis-neodecanoate diaminocyclohexane platinum liposomes (Aroplatin) | Phase-2 | Colorectal cancer |
Lurtotecan liposomes (OSI-211) | Phase-2 | Ovarian cancer |
SN38 liposomes (LE-SN38) | Phase-2 | Colorectal cancer |
Paclitaxel liposomes (LEP-ETU) | Phase-2 | Breast cancer |
Annamycin liposomes | Phase-2 | Acute lymphocytic leukemia |
Tretinoin liposomes (Atragen) | Phase-2 | Kidney cancer |
Doxorubicin liposomes (glutathione Pegylated) (2B3101) | Phase-2 | Solid tumors and brain cancer |
Protein Kinase N3 siRNA liposomes (Atu027) | Phase-2 | Pancreatic cancer |
p53 plasmid liposomes (SGT-53) | Phase-2 | Pancreatic cancer, glioblastoma |
SN38 micelles (NK012) | Phase-2 | Breast cancer |
Paclitaxel liposomes (LEP-ETU) | Phase-2 | Breast cancer |
Annamycin liposomes | Phase-2 | Acute lymphocytic leukemia |
Tretinoin liposomes (Atragen) | Phase-2 | Kidney cancer |
Doxorubicin liposomes (glutathione Pegylated) (2B3101) | Phase-2 | Solid tumors and brain cancer |
Protein Kinase N3 siRNA liposomes (Atu027) | Phase-2 | Pancreatic cancer |
p53 plasmid liposomes (SGT-53) | Phase-2 | Pancreatic cancer, glioblastoma |
SN38 micelles (NK012) | Phase-2 | Breast cancer |
Doxorubicin micelles (SP1049C) | Phase-2 | Advanced esophagus or gastroesophageal junction adenocarcinoma |
Docetaxel micelles (CPC634) | Phase-2 | Ovarian cancer |
Categories
Internal Stimuli Responsive drugs :
As presented in the definition part, internal stimuli responsive drugs refers to drugs made with biomaterials or nanocarriers having the ability to respond specific triggers located in their micro-environment. These triggers can be physiological ( enzyme, redox, thermic …) or linked to the specific pathology targeted ( Xiao Fu et al. 2018).
Enzyme-responsive drug delivery system :
Enzyme-responsive DDS refers to system that has modification of physicochemical properties after enzymatic actions, this may lead to the liberation of the active agents and obtention of the therapeutic effect wanted (Sabya Sachi et al., 2020). Many enzymes like lipase, protease, trypsin, glycosidase, phospholipase, oxidoreductase etc. are selected in this therapeutic strategy and for several pathologies such as cancer therapies ( Nguyen et al. 2015). The process is simple and can be summarized on the picture below
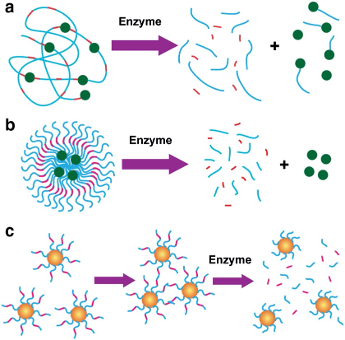
Figure 4 Enzyme-responsive nanomaterials for drug delivery and diagnostics; a) polymer-based nanoparticles can be covalently modified with drugs through an enzyme-cleavable linker so that the enzyme activity triggers drug delivery in the tissue of interest; b) polymer-stabilized liposomes can be loaded with drugs, whose degradation can be programmed to be triggered by an enzyme. c) Inorganic particles can be used for diagnostic when the activity of the target hydrolase controls the assembly or disassembly of the nanoparticles, which turn in changes the nanoparticle solution
Here are several uses of enzymes-responsive technologies in DDS :
- MPP-Responsive, polymeric systems
High matrix metalloproteinases (MMPSs) concentrations have been identified and associated with many cancerous cells. This special type of enzyme could selectively cleave peptide linkages in between nonterminal amino acid sequences ( Van Rijt S. H. et al. 2015). MMP2-sensitive siRNA delivering system development, with self-assembling and copolymeric technology of the polyethylene glycol-peptide-polyethylenimine-12, along with dioleoyl-snglycero-3-phosphoethanolamine (PEG-pp-PEI-PE), is referred as one of the latest innovation. The linker was based on an octapeptide, GPLGIAGQ, which is high sensitive to MMP2 with big response. From this process high tumor targeting of the developed system merged (Zhu L. et al. 2014). A different study showed the use of N-(3-aminopropyl) methacrylamide (APM) and acrylaminde (AAM) to synthesize copolymeric nanocapsules with MMP-responsive peptide cross-linkers to cargo BSF and VEGF (Wen J. et al, 2014). The design of novel MMP2 sensitive nanoparticles were used in a recent work, these designed from copolymeric TPGS3350-pp-PLA along with TPGS-folate to deliver anticancer drugs (Pan J. et al, 2018). An MMP 8-responsive, polymeric hydrogel was developed from a diacrylate-containing polyethylene glycol-based moiety, along with a cysteine-terminated peptide cross-linker (CGPQG↓IWGQC). The encapsulation system used for the hydrogels was made with , and antibacterial peptide KSL and they were used to target chronic periodontitis and peri-implantitis (Guo J. et al, 2019).
- Esterase-responsive, polymeric systems
This system relies on the ester bond cleavage to the targeted drug Several esterase-responsive, polymeric systems have been developed lately by researchers, we will present some here. The development of the PAMAM-based, polymeric dendrimer is focused to his link to PTX via succinate bond. This PTX/PAMAM G4 dendrimers were readily hydrolyzed by esterase and then release the free drug, this system helps an optimization of internalization in effective cell and toxicity reduction. Upon conjugation with PEG, these systems revealed an enhanced action which could be explored to develop more anticancer drugs ( Khandare J.J. et al., 2006). For pathologies such as inflammation, a novel twin-drug system, i.e., Dexamethasone-Diclofenac, merged with a system including esterification reaction and then encapsulated into polylactide (PLA) nanoparticles. In vivo studies of the were done using esterase systems. Results shows that esterase hydrolysis enhanced the drug release rate, and the synergistic activity of the two anti-inflammatory drugs led to higher inhibition of the TNF-α level than the free drug ( Assali M. et al., 2018).
- Cathepsin B.
The use of the tetrapeptide linkage Gly-Phe-Leu-Gly is one of the common viewed in the enzyme-responsive design, polymeric systems, this is justified by the easy cleavage by cathepsin B, an lysosomal enzyme with expression in tumor tissues (GIANASI E. ET AL, 2002). The development of a PEGylated gemcitabine-containing with cathepsin B responsive action was made up to target tumor microenvironments (Zhang C. , 2017). Another study with a peptide macromonomer was designed from BIM and cathepsin B substrate, which was then incorporated into copolymeric deblock system of DEAEMA/BMA and DEAEMA. This shown a great and promising result in the intracellular delivery of peptides (Kern H. B. et al, 2017).
Swelling controlled drug delivery system :
“Swelling-controlled drug delivery system” refers to therapeutical devices where the release is controlled by the swelling step. This can also be used for devices where the swelling step has a high importance or plays an important role on the mass transport process. (e.g., drug dissolution, drug diffusion, and polymer dissolution) (Siepmann J. et al., 2012).
Most of swelling-controlled DDS are based on hydrophilic polymers like the hydroxypropyl methylcellulose ( Colombo P. et al, 2008; Escudero JJ et al, 2010). We can see on the figure 5 the process on the polymer swelling. From dry state (non-swollen state) where the polymer has a high density and the mobility of the macromolecules is restricted to the swollen state where due to hydratation we can see a relaxation on the polymer chains and increase of volume and mobility of the macromolecules. Thus the physical changes on the polymer drives the control of the release rate of the drug.
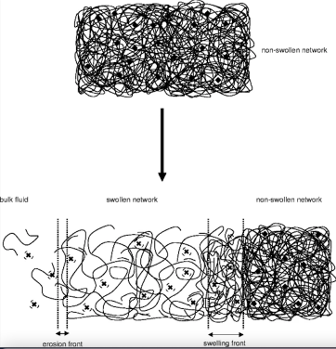
pH-responsive drug delivery system :
pH is a major factor regarding the DDS for any drug administrated into the body, the delivery of a drug must manage that the physiological needs at the targeted sites matches deeply to the different organs targeted and the tissues or cellular compartment properties. Thus pH is a great parameter on Stimuli responsive drugs and other DDS. The dependency of the therapeutic efficacity to the control in time and location of delivery are one of the points raised by this DDS (Zhu YJ. Et al, 2015).
Under normal physiological states, pH varies on different sites and remains more or less stale depending on the location site and the organs (table 2).
Table 2 pH values in physiological conditions (Yongxu Mu et al. 2021)
Site | pH value |
Plasma | 7,38 – 7,42 |
Saliva | 6,0 – 7,0 |
Gastric Juice | 1,0 – 3,5 |
Bile | 7,8 |
Pancreatic juice | 8,0 – 8,3 |
Small Intestinal fluid | 5,5 – 7,0 |
Lysosome | 4,5 – 5,0 |
Golgi Apparatus | 6,0 – 6,7 |
Different strategies are used on the pH responsive DDS, depending on the material used and the targeted sites; literature divided them into four big responses modes ( Yongxu Mu et al., 2021) :
- physical dissociation
- chemical bond cleavage -resulting nanoparticle dissociation
- chemical bond cleavage promoting payload release
- nanoparticle swelling
The process can easily be observed on the figure bellow
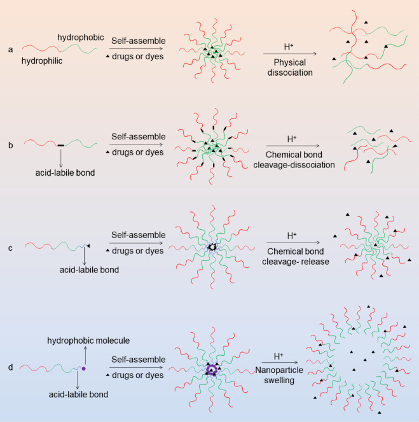
Redox-Responsive DDS :
The use of Redox-responsive DDS is mostly for polymer technologies. In this process the disulfide is cleaved by a high concentration of GSH, an abundant thiol present in cell cytoplasm. Its function is mostly reduction in biochemical processes ( Thambi et al., 2016). GSH concentrations varies deeply depending on the tissue where they’re present (normal or pathological) and the pathological state of the patient. In some pathologies like neurodegenerative diseases, liver diseases, stokes, seizures or diabetes we can have an increase rate of GSH (Estrella et al., 2006). Thus this difference of redox in the environment is also present in tumor cells and is definitely useful to build up DDS based on redox-responsive nanomaterials (Schafer et al., 2001).
Here is a figure representing the use of nanocarriers in the redox-responsive mechanism ( (figure7).
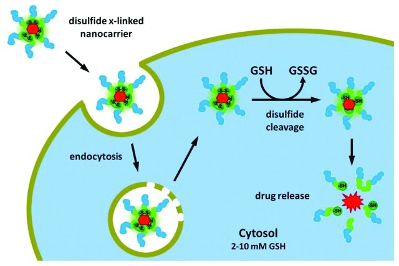
Thermo-Responsive drugs :
Thermo-responsive system are mostly used in DDS for therapeutical strategies; they can be used for both diagnostic and treatment (Son H. et al; 2020). Mostly dehydration is the main process leading to drug release on the thermos-sensitive process in DDS. The NCs response to the external temperature change modify the polymer chain and this can then lead to drug release (Liu et al., 2016). Mostly tumor cells do have a higher temperature ( 40 – 42°C ) than normal cells (37°C). Thus the release of the payload is obtained by the contact of the specified tissues with higher temperature ( Liu et al., 2016).
Two different approaches can be used for DDS with thermos-responsive NCs :
- NCs responsive to high temperature drug release :
We can have for example in this part the polycaprolactone ( N-Isopropyl acrylamide) (PNIPAM) which displays tunable properties and a reduction of the LCST with an increase of the PAA content. Its use can be applied to various switching of DDS where he responses to small pH changes. Their use showed a cell viability of 20% at 40°C versus a viability of 40% at 37°C for treatments using this technology ( Son H. et al.; 2020).
The second approach involving external stimuli of higher temperature will be developed on the following point.
Glucose-triggered drug delivery system :
The use of hydrogels in DDS and other therapeutic applications as soft biomaterials have increased lately in different applications. The deep resemblance to hydrogels and the extracellular matrix (ECM) conditions plus their ability to load the drugs and other bioactive molecules made hydrogels promising candidates for the development of smart DDS (Allan S. Hoffman, 2012). Hydrogels that respond to specific stimuli have attracted the attention of researchers because of their potential for space, temporal and ondemand delivery options. Their use can be sorted in different ranges of application from sensing, biotech to tissue engineering ( Heidi R. Culver et al., 2017). The research groups of Chang-Li and Xiaoyu Liu showed dual-sensitive nanogels that can be used in improvement of oxidation-responsive and glucose triggered hydrogels for insulin delivery (Li C et al., 2019).
The same type of preparation using the oxidation-responsive hydrogels used a four-arm polyethylene glycol (PEG) containing reactive oxygen cleavable acrylic bonds supported by phenylboronic ester pendant groups was used in M. Zang work (Figure 8.a). These hydrogels were formed mostly with hydrogels hydrophilic PEG and a fraction of hydrophobic linker molecules. Their mechanical strength was an asset to a macroscopic gel structure under culture conditions, and less toxicity appeared with LO2 cells in vitro. Cleavage of hydrogels and the release of payloads is achieved only in the presence of reactive oxygen stimuli such as hydrogen peroxide (H2O2). Glucose oxidase (GOx), is an enzyme causing glucose’s oxidation to gluconolac-tone and produces H2O2 is encapsulated into the hydrogels. (Shivaprasad M. et al., 2018). GOx incorporation has bestowed the hydrogels with a glucose responsive nature. The use of hydrogels with GOx and FITC-insulin is tremendous in the demonstration og glucose triggered release of insulin under in vitro conditions. The release of encapsulated insulin (FITC-insulin) in the presence of external H2O2 and glucose was monitored using fluorescence measurements and the loss of gel integrity was measured by rheology and visual disintegration of the hydrogels (Figure 8.b). The release of encapsulated insulin was found to be dependent on the concentration of triggering molecules used (either H2O2 or glucose) and a faster release of insulin along with degradation of hydrogels was observed at high concentrations of stimuli molecules. Strikingly, the GOx encapsulated hydrogels were found to be responsive to the pathological glu- cose concentration (20 μM) and were triggered to release the insulin under in vitro conditions(Shivaprasad M. et al., 2018).
GOx encapsulated oxidation sensitive PEG hydrogels have showed several properties as high selectivity, sensitivity and cytocompatibility this ended up with hydrogels giving temporal on-demand release of insulin with monitoring of insulin release depending on the needs. These strategies can be used in several diabetics cases as foot ulcers. The ability of hydrogels to release payloads in the presence of H2O2 stimuli further extends the suitability in the treatment of ROS associated diseases and tissue engineering.
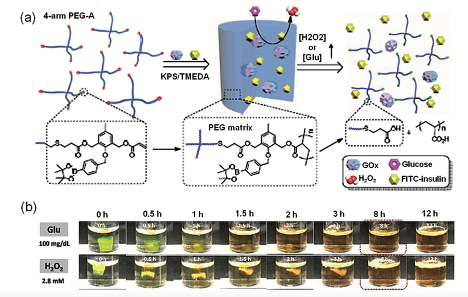
External Stimuli Responsive drugs :
Thermo-responsive Nanomedicines drugs :
The second approach used in DDS with thermo-responsive NCs is the use of carriers that burst drug release in response to external triggers increasing the temperature in the drug carrier. This alters the thermo-sensitive DDS and end-up to a drug release on the targeted site (Khoee S. et al., 2018). This process may include for example PPy in amphiphilic di-block copolymer for tumor treatment. PPy has a photothermal property which leads to the promotion of drug release from the micelles (Son H. et al; 2020).
Magnetic-responsive Nanomedicines drugs
Lately the use of magnetic-responsive materials in the microelectronics, biomedical applications, microfluidics and chemistry fields increased deeply. Magnetism is an external non-invasive method of activation that has attractive capabilities because it can be controlled in a temporal and spatial (Mahdi Karimi et al., 2015). The use of DDS with Magnetic stimuli response are considered as best option for the design of efficient drug delivery and lesser interaction than some other stimuli responsive DDS,e.g. pH, ultrasound and light (Mophatra A. et al., 2019).
Photo-responsive DDS :
Several elements as non-invasiveness, inexpensiveness, and practicability leaded to the use of light interest for DDS as an exogenous stimulus. Its use for prodrug-based nanomedicines as an external stimuli gave many advantages over other internal stimuli following the ease of handling, precise control of the time and location of treatment ( Mura S. et al, 2013). The use of light-responsive smart prodrug-based nanosystems using ultraviolet (UV), visible light, and near-infrared (NIR) ( figure 9.) have been intensively applied for non-invasive and controlled on-demand drug release against cancer (Schroeder A. et al, 2012 ; Yang G. et al,2016 ; Jia S. et al., 2018).
Due to the consequences on structural transitioning modifications with UV light (300-380nm) on polymers with special structures ( as O-nitrobenzyl, pyrene, spiropyran, and azobenzene), it is widely used in prodrug release ( Jia S. et al, 2018 ; Azagarsamy M.A. et al, 2012 ; Tong R. et al, 2012). We do have for example Liu’s group work using a photocaged linkage to form prodrugs-based shell cross-linked (SCL) which were composed of P(CL-g-CPT)-b-P(OEGMA-co-MAEBA)-CPT and PCL-b-P(OEGMA-co-MAEBA-co-FA) amphiphilic diblock copolymers. This using a photocaged CPT prodrug enhancing cleavage and drug release on the micelles under UV irradiations ( Angel Xie et al., 2020).
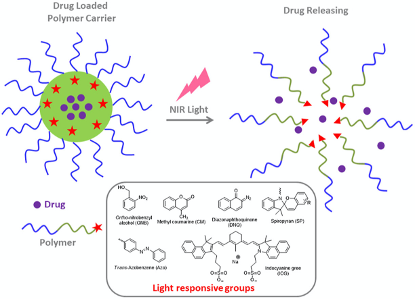
Some Materials used in DDS :
The huge demand for adapted and innovative processes in DDS raised more interests than ever in SRD and implementation of latest improvement in drug engineering and nanotechnologies moved to the next generation of drugs with less side effects and huge efficiency.
In fact different materials are used in SRD formulation, depending on their payload and the specific receptors targeted; It’s important for this process to keep stability through the delivery process and maximum reduction of exogeneous interactions ( food, poly-medication, other medical tools etc.).
We will focus on the type of stimuli to categorize the different SRD, thus we have :
- pH responsive DDS
Depending on the type of disease, there is a wide range of materials pH-sensitive that can be used in DDS. The engineering of hydrogels for their physicochemical properties as stiffness, degradation and porosity helps to directly act with influence on the fates of the encapsulated cells including their migration, proliferation, differentiation, and communication. Furthermore, stimuli-responsive hydrogels could improve the in vivo therapeutic efficacy and also provide new therapeutic pathways ( Wang L. et al., 2018).
Thermos-responsive materials
- Light-responsive materials
- Redox-responsive materials and electroactive polymers
- Magnetic-responsive nanomaterials
Challenges in DDS :
In conclusion, the nano-tech aided engineering novel polymeric carriers are hugely growing in various types of drug delivery applications. With key scientific advances, massive research efforts are being made with a particular focus to construct DDS that can respond to the physiochemical-based surrounding changes that are either external or internal in a sophisticated manner with high-level control and precision. In this paper, we summarized the uniqueness of stimuli-responsive polymeric carriers and their potentialities for targeted drug delivery applications. Considering suitable examples, an array of stimuli-responsive DDS, for instance, redox-responsive, pH-responsive, temperature-responsive, photo/light-responsive, magnetic-responsive, ultrasound-responsive, and electrical-responsive DDS, and/or all-in-all dual/dual/multi-responsive DDS (combination or two or more from any of the above) have been discussed. For easy understanding and to highlight the work behavior of different DDS, schematic illustrations are also included by giving the focus on chemistry aspects, release behavior, and drug delivery applications. Aside from the significant advancement in the biomedical/pharmaceutical at large and DDS arena, in particular, there are still many concerns that remain to be addressed. For instance, biocompatibility, biodegradability, non-toxicity, and safer elimination of the smart carriers from the biological system are some of the significant limiting factors that one needs to be considered prior to designing DDS. Furthermore, to induce fast responsiveness, the size of the carrier also plays a critical role. Nanosized carriers are being developed with comprisable mechanical characteristics, which is one of the important features required for biomedical-based applications. Besides processing drawbacks, administrative issues to get approval to use the designed polymeric-based smart DDS is another major bottleneck in the field. To avoid all these limitations, future investigations should be directed to introduce novel technologies to design DDS using polymeric materials with inherent properties such as biocompatibility, biodegradability, and non-toxicity. In conclusion, many polymer-based stimuli-responsive carriers offer substantial potential for biomedical applications. Thus, the external/internal stimuli-responsive carriers hold great promise for nanomedicine in the coming future.
Discussion :
Stimuli-responsive materials are a smart choice for the development of DDS meanwhile their use in pharmaceutical industry required adaptability and efficiency in the different targeted pathologies. The use of these materials the past years improved in several therapies as tumors, chronic inflammation, diabetes and even diagnosis or anesthesia procedures.
Access to a plethora of physical, chemical and biological stimuli have resulted in the design and development of smart drug delivery systems with controlled spatiotemporal drug delivery ( Aida Lopez et al., 2022).
In our work we discussed a few selected examples of stimuli DDS based on pH, glucose, enzyme, light, magnetic field and ultrasound triggers under in vitro conditions, and an example of NIR-triggered in vivo used in photo responsive DDS.
Despite great progresses observed in smart drug delivery systems for various diseases using strategies with diverse stimuli triggers, there is still a need for next generation stimuli-responsive drug delivery systems and devices with more precise and on-demand delivery. Importantly, most of the reported stimuli-responsive drug delivery systems and devices are tested under either in vitro or in cellulo settings, but not under in vivo conditions involving real pathological situations and also interactions with several exogenous components as food or other drugs co-administrated to patients. In spite of the recent progress in stimuli-respon- sive smart delivery devices, there is a plenty of room for the development of next-generation multifunctional smart drug delivery systems and devices that are biocompatible and biodegradable, in addition to being spatiaotem- poral, target specific, for on-demand delivery.
Bibliography
- Alkilani, A.Z.; McCrudden, M.T.C.; Donnelly, R.F. Transdermal Drug Delivery: Innovative Pharmaceutical Developments Based on Disruption of the Barrier Properties of the Stratum Corneum. Pharmaceutics 2015, 7, 438-470.
- Allan S. Hoffman, Hydrogels for biomedical applications, Advanced Drug Delivery Reviews, Volume 64, Supplement, 2012, Pages 18-23, ISSN 0169-409X, https://doi.org/10.1016/j.addr.2012.09.010.
- B. Sana, A. Finne-Wistrand, D. Pappalardo, Recent development in near infrared light-responsive polymeric materials for smart drug-delivery systems, Materials Today Chemistry, Volume 25, 2022, 100963, ISSN 2468-5194,
- C. Huang, et al. Synthesis of polyhalo acridones as pH-sensitive fluorescence probes Bioorg. Med. Chem. Lett., 20 (15) (2010), pp. 4665-4669
- CBIP 2022 updates
- Colombo P, Santi P, Siepmann J, Colombo G, Sonvico F, Rossi A, Strusi OL (2008) Swellable and rigid matrices: controlled release matrices with cellulose ethers. In: Augsburger LL, Hoag SW (eds) Pharmaceutical dosage forms: tablets, vol 2: rational design and formulation, 3rd edn. Informa Healthcare, New York
- Cui D., Huang J., Zhen X. et al. A semiconducting polymer nano-prodrug for hypoxia-activated photodynamic cancer therapy. Angew Chem Int Ed. 2019; 58: 5920-5924
- Culver HR, Clegg JR, Peppas NA. Analyte-Responsive Hydrogels: Intelligent Materials for Biosensing and Drug Delivery. Acc Chem Res. 2017 Feb 21;50(2):170-178. doi: 10.1021/acs.accounts.6b00533. Epub 2017 Feb 7. Erratum in: Acc Chem Res. 2018 Oct 16;51(10):2600. PMID: 28170227; PMCID: PMC6130197.
- Dai Y., Xiao H., Liu J., et al. In Vivo multimodality imaging and cancer therapy by near-infrared light-triggered trans-platinum pro-drug-conjugated upconverison nanoparticles. J Am Chem Soc. 2013; 135: 18920
- Escudero JJ, Ferrero C, Jimenez-Castellanos MR (2010) Compaction properties, drug release kinetics and fronts movement studies from matrices combining mixtures of swellable and inert polymers. II. Effect of HPMC with different degrees of methoxy/hydroxypropyl substitution. Int J Pharm 387:56–64
- Fleige E, Quadir MA, Haag R. Stimuli-responsive polymeric nanocarriers for the controlled transport of active compounds: concepts and applications. Adv Drug Deliv Rev. 2012 Jun 15;64(9):866-84. doi: 10.1016/j.addr.2012.01.020. Epub 2012 Feb 11. PMID: 22349241.
- Freya Q. Schafer, Garry R. Buettner, Redox environment of the cell as viewed through the redox state of the glutathione disulfide/glutathione couple, Free Radical Biology and Medicine, Volume 30, Issue 11, 2001, Pages 1191-1212, ISSN 0891-5849, https://doi.org/10.1016/S0891-5849(01)00480-4.
- Gaudana, R., Ananthula, H.K., Parenky, A. et al. Ocular Drug Delivery. AAPS J 12, 348–360 (2010). https://doi.org/10.1208/s12248-010-9183-3
- Gianasi E., Buckley R.G., Latigo J., Wasil M., Duncan R. HPMA copolymers platinates containing dicarboxylato ligands. Preparation, characterisation and in vitro and in vivo evaluation. J. Drug Target. 2002;10:549–556. doi: 10.1080/1061186021000072456.
- Guan X., Chen Y., Wu X., Li P. and Liu Y. Enzyme-responsive sulfatocyclodextrin/prodrug supramolecular assembly for controlled release of anti-cancer drug chlorambucil Chem Commun. 2019; 55: 953-956 https://doi.org/10.3390/pharmaceutics7040438
- Ishwor Poudel, Manjusha Annaji, Robert D. Arnold, Virendra Gajbhiye, Amit K. Tiwari, R. Jayachandra Babu, Chapter 3 – Vesicular nanocarrier based stimuli-responsive drug delivery systems, Editor(s): Virendra Gajbhiye, Kavita R. Gajbhiye, Seungpyo Hong, Stimuli-Responsive Nanocarriers, Academic Press, 2022, Pages 61-86, ISBN 9780128244562,
- Jia R, Teng L, Gao L, Su T, Fu L, Qiu Z, Bi Y. Advances in Multiple Stimuli-Responsive Drug-Delivery Systems for Cancer Therapy. Int J Nanomedicine. 2021 Feb 25;16:1525-1551. doi: 10.2147/IJN.S293427. PMID: 33658782; PMCID: PMC7920594.
- Jia S., Fong W.-.K., Graham B., Boyd B.J Photoswitchable molecules in long-wavelength light-responsive drug delivery: from molecular design to applications. Chem Mater. 2018; 30: 2873-2887
- Jinsong Hao & Paul W. S. Heng (2003) Buccal Delivery Systems, Drug Development and Industrial Pharmacy, 29:8, 821-832, DOI: 10.1081/DDC-120024178
- Karim Amighi, Galenic Pharmacy course PHARJ404, Academic Year 2020 – 2021
- Kern H.B., Srinivasan S., Convertine A.J., Hockenbery D., Press O.W., Stayton P.S. Enzyme-Cleavable Polymeric Micelles for the Intracellular Delivery of Proapoptotic Peptides. Mol. Pharm. 2017;14:1450–1459. doi: 10.1021/acs.molpharmaceut.6b01178.
- Khandare J.J., Jayant S., Singh A., Chandna P., Wang Y., Vorsa N., Minko T. Dendrimer versus linear conjugate: Influence of polymeric architecture on the delivery and anticancer effect of paclitaxel. Bioconjugate Chem. 2006;17:1464–1472. doi: 10.1021/bc060240p.
- Khoee, S.; Karimi, M.R. Dual-Drug loaded Janus graphene oxide-based thermoresponsive nanoparticles for targeted therapy. Polymer 2018, 142, 80–98
- Labiris NR, Dolovich MB. Pulmonary drug delivery. Part I: physiological factors affecting therapeutic effectiveness of aerosolized medications. Br J Clin Pharmacol. 2003 Dec;56(6):588-99. doi: 10.1046/j.1365-2125.2003.01892.x. PMID: 14616418; PMCID: PMC1884307.
- Laura Elizabeth Lansdowne, Technology Networks / Drug Discovery, December 2020
- Li C , Liu X , Liu Y , Huang F , Wu G , Liu Y , Zhang Z , Ding Y , Lv J , Ma R , An Y , Shi L . Glucose and H2O2 dual-sensitive nanogels for enhanced glucose-responsive insulin delivery. Nanoscale. 2019 May 9;11(18):9163-9175. doi: 10.1039/c9nr01554j. PMID: 31038150.
- Ling X., Tu J., Wang J. et al. Glutathione-Responsive Prodrug Nanoparticles for Effective Drug Delivery and Cancer Therapy. ACS Nano. 2019; 13: 357-370
- Liu D, Yang F, Xiong F, Gu N. The Smart Drug Delivery System and Its Clinical Potential. Theranostics. 2016 Jun 7;6(9):1306-23. doi: 10.7150/thno.14858. PMID: 27375781; PMCID: PMC4924501.
- Luo C, Sun J, Sun B, He Z. Prodrug-based nanoparticulate drug delivery strategies for can- cer therapy. Trends Pharmacol Sci 2014;35(11):556–66.
- M. Kanamala, W.R. Wilson, M. Yang, B.D. Palmer, Z. Wu Mechanisms and biomaterials in pH-responsive tumour targeted drug delivery: a review Biomaterials, 85 (2016), pp. 152-167
- Mahdi Karimi, Parham Sahandi Zangabad, Amir Ghasemi and Michael R Hamblin
Smart External Stimulus-Responsive Nanocarriers for Drug and Gene Delivery Smart External Stimulus-Responsive Nanocarriers for Drug and Gene Delivery chapter 4 Magnetic-responsive nanocarriers; Published November 2015 • Copyright © 2015 Morgan & Claypool Publishers Pages 4-1 to 4-6 - McDaniel J.R., Bhattacharyya J., Vargo K.B. et al. Self-assembly of thermally responsive nanoparticles of a genetically encoded peptide polymer by drug conjugation. Angew Chem Int Ed. 2013; 52: 1683-1687
- Mohapatra A, Fujiwara T, Harris MA, LeVine DA, Ghimire M, Morshed BI, Jennings JA, Bumgardner J, Haggard WO, Mishra SR. Magnetic Stimulus Responsive DDS Based on Chitosan Microbeads Embedded with Magnetic Nanoparticles. Annu Int Conf IEEE Eng Med Biol Soc. 2019 Jul;2019:1674-1677. doi: 10.1109/EMBC.2019.8857406. PMID: 31946218.
- Nguyen M.M., Carlini A.S., Chien M.-P., Sonnenberg S., Luo C., Braden R.L., Osborn K.G., Li Y., Gianneschi N.C., Christman K.L. Enzyme-Responsive Nanoparticles for Targeted Accumulation and Prolonged Retention in Heart Tissue after Myocardial Infarction. Adv. Mater. 2015;27:5547–5552. doi: 10.1002/adma.201502003
- Pan J., Li P.J., Wang Y., Chang L., Wan D., Wang H. Active targeted drug delivery of MMP-2 sensitive polymeric nanoparticles. Chem. Commun. 2018;54:11092–11095. doi: 10.1039/C8CC05504A.
- Pham, S.H.; Choi, Y.; Choi, J. Stimuli-Responsive Nanomaterials for Application in Antitumor Therapy and Drug Delivery. Pharmaceutics 2020, 12, 630. https://doi.org/10.3390/pharmaceutics12070630
- Prausnitz, M., Mitragotri, S. & Langer, R. Current status and future potential of transdermal drug delivery. Nat Rev Drug Discov 3, 115–124 (2004). https://doi.org/10.1038/nrd1304
- Rahim MA, Jan N, Khan S, Shah H, Madni A, Khan A, Jabar A, Khan S, Elhissi A, Hussain Z, Aziz HC, Sohail M, Khan M, Thu HE. Recent Advancements in Stimuli Responsive Drug Delivery Platforms for Active and Passive Cancer Targeting. Cancers (Basel). 2021 Feb 7;13(4):670. doi: 10.3390/cancers13040670. PMID: 33562376; PMCID: PMC7914759.
- Sankha Bhattacharya, Md Meraj Anjum, Krishna Kumar Patel. (2022) Gemcitabine cationic polymeric nanoparticles against ovarian cancer: formulation, characterization, and targeted drug delivery. Drug Delivery 29:1, pages 1060-1074.
- Schroeder A., Goldberg M.S., Kastrup C. et al. Remotely activated protein-producing nanoparticles. Nano Lett. 2012; 12: 2685-2689
- Shaffer, S.A., Baker-Lee, C., Kennedy, J. et al. In vitro and in vivo metabolism of paclitaxel poliglumex: identification of metabolites and active proteases. Cancer Chemother Pharmacol 59, 537–548 (2007). https://doi.org/10.1007/s00280-006-0296-4
- Siepmann, J., Siepmann, F. (2012). Swelling Controlled Drug Delivery Systems. In: Siepmann, J., Siegel, R., Rathbone, M. (eds) Fundamentals and Applications of Controlled Release Drug Delivery. Advances in Delivery Science and Technology. Springer, Boston, MA. https://doi.org/10.1007/978-1-4614-0881-9_7
- Siepmann, J., Siepmann, F. (2012). Swelling Controlled Drug Delivery Systems. In: Siepmann, J., Siegel, R., Rathbone, M. (eds) Fundamentals and Applications of Controlled Release Drug Delivery. Advances in Delivery Science and Technology. Springer, Boston, MA. https://doi.org/10.1007/978-1-4614-0881-9_7
- Sun B., Luo C., Yu H. et al. Disulfide bond-driven oxidation- and reduction-responsive prodrug nanoassemblies for cancer therapy. Nano Lett. 2018; 18: 3643-3650
- Thambi, T., Park, J. H., and Lee, D. S. (2016a). Stimuli-responsive polymersomes for cancer therapy. Biomater. Sci. 4, 55–69.
- V.P. Torchilin. Fundamentals of stimuli-responsive drug and gene delivery systems, 2018.
- Van Rijt S.H., Bolukbas D.A., Argyo C., Datz S., Lindner M., Eickelberg O., Konigshoff M., Bein T., Meiners S. Protease-mediated release of chemotherapeutics from mesoporous silica nanoparticles to ex vivo human and mouse lung tumors. ACS Nano. 2015;9:2377–2389. doi: 10.1021/nn5070343.
- Wells CM, Harris M, Choi L, Murali VP, Guerra FD, Jennings JA. Stimuli-Responsive Drug Release from Smart Polymers. J Funct Biomater. 2019 Jul 31;10(3):34. doi: 10.3390/jfb10030034. PMID: 31370252; PMCID: PMC6787590.
- Wen J., Anderson S.M., Du J., Yan M., Wang J., Shen M., Lu Y., Segura T. Controlled protein delivery based on enzyme-responsive nanocapsules. Adv. Mater. 2011;23:4549–4553. doi: 10.1002/adma.201101771.
- Woolfson AD, Malcolm RK, Gallagher R. Drug delivery by the intravaginal route. Crit Rev Ther Drug Carrier Syst. 2000;17(5):509-55. PMID: 11108158.
- Xiao Fu, Leticia Hosta-Rigau, Rona Chandrawati, Jiwei Cui, Multi-Stimuli-Responsive Polymer Particles, Films, and Hydrogels for Drug Delivery, Chem, Volume 4, Issue 9, 2018, Pages 2084-2107,
- Xu X., Saw P.E., Tao W. et al. ROS-responsive polyprodrug nanoparticles for triggered drug delivery and effective cancer therapy. Adv Mater. 2017; 291700141
- Yang G, Xu L, Chao Y, et al. Hollow MnO2 as a tumor-microenvironment-respon- sive biodegradable nano-platform for combination therapy favoring antitumor immune responses. Nat Commun 2017;8(1):902.
- Yang G., Liu J., Wu Y., Feng L., Liu Z Near-infrared-light responsive nanoscale drug delivery systems for cancer treatment. Coord Chem Rev. 2016; 320: 100-117
- Yongxu Mu, Lidong Gong, Tianjiao Peng, Jun Yao, Zhiqiang Lin, Advances in pH-responsive drug delivery systems, OpenNano, Volume 5, 2021, 100031, ISSN 2352-9520, https://doi.org/10.1016/j.onano.2021.100031.
- Zhang Y., Teh C., Li M. et al. Acid-Responsive Polymeric Doxorubicin Prodrug Nano-particles Encapsulating a Near-Infrared Dye for Combined Photothermal-Chemotherapy. Chem Mater. 2016; 28: 7039-7050
- Zhang, H., Zhang, J. & Streisand, J.B. Oral Mucosal Drug Delivery. Clin Pharmacokinet 41, 661–680 (2002). https://doi.org/10.2165/00003088-200241090-00003
- Zhu L., Perche F., Wang T., Torchilin V.P. Matrix metalloproteinase 2-sensitive multifunctional polymeric micelles for tumor-specific co-delivery of siRNA and hydrophobic drugs. Biomaterials. 2014;35:4213–4222. doi: 10.1016/j.biomaterials.2014.01.060
- Zhu YJ, Chen F. pH-responsive drug-delivery systems. Chem Asian J. 2015 Feb;10(2):284-305. doi: 10.1002/asia.201402715. Epub 2014 Oct 9. PMID: 25303435.
- Wang, L.; Neumann, M.; Fu, T.; Li, W.; Cheng, X.; Su, B.-L. Porous and responsive hydrogels for cell therapy. Curr. Opin. Colloid Interface Sci. 2018, 38, 135–157.